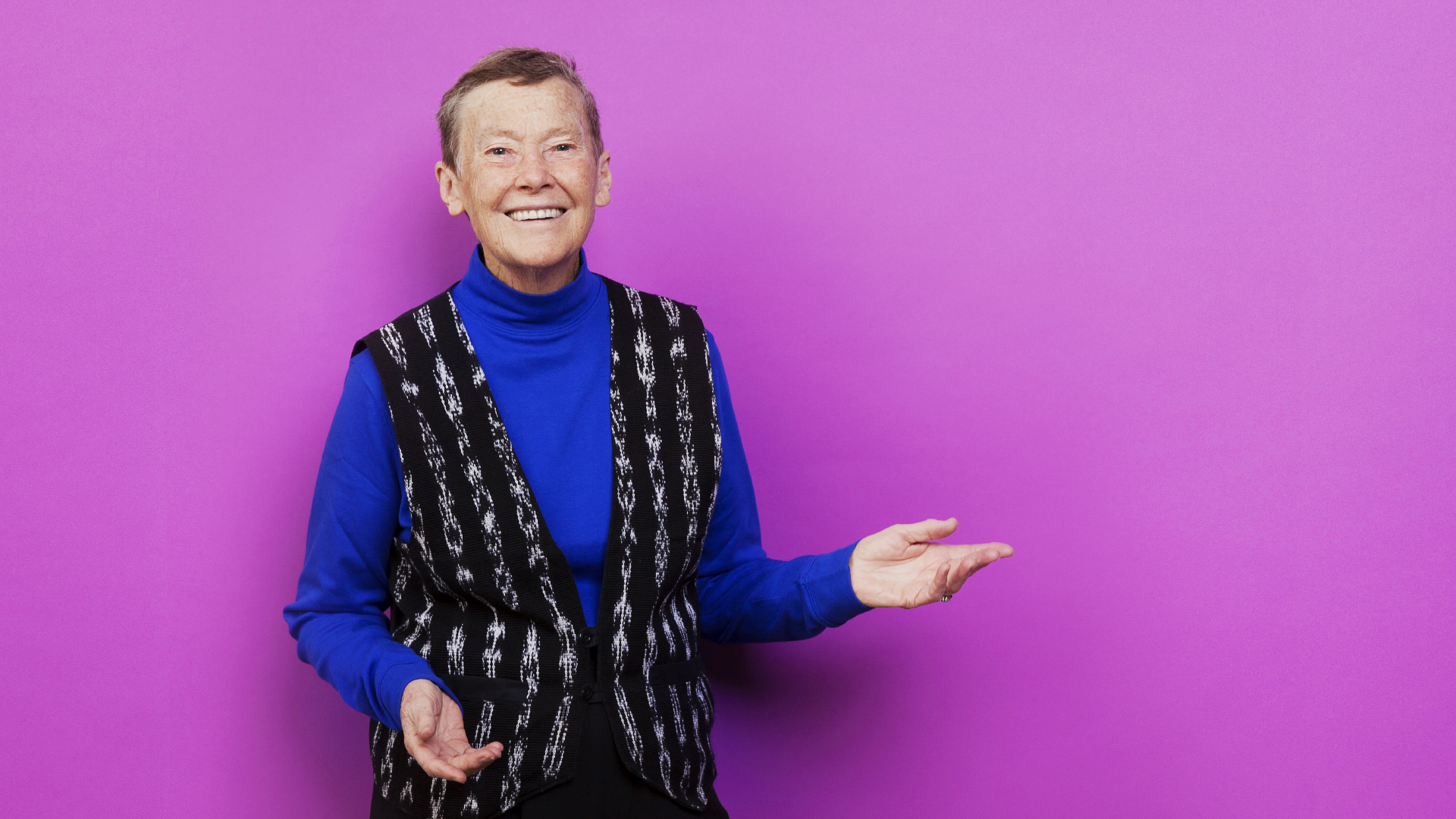
Peter DaSilva for Quanta Magazine
Introduction
Before Helen Quinn was a famous theoretical physicist, she thought about becoming a teacher. Now, in the second act of her career, she has come full circle, helping to craft the Next Generation Science Standards, which have been adopted by 17 states plus the District of Columbia. But her path to becoming both a world-class physicist and a leader of science education reform was one she almost didn’t take.
Quinn, who is now 73, grew up in Australia, where she had to decide on an academic focus by her sophomore year in high school. Her father was an engineer, and family conversations often revolved around how things work. “The kind of problem solving that I recommend as useful for learning science was part of our family culture,” she said.
She recalled how a high school teacher encouraged her to become a mathematician, telling her, “Because you’re so lazy, you will never solve a problem the hard way. You always have to figure out a clever way.” But in the 1950s, she said, “the idea that a woman could be an engineer was nonexistent. I once walked into the engineering school at the University of Melbourne, and one guy said, ‘Look what’s got in here,’ and the other one says, ‘You think it’s real?’”
After Quinn transferred to Stanford University in 1962, her adviser encouraged her to consider graduate school, even though, as he explained, “graduate schools are usually reluctant to accept women because they get married and they don’t finish. But I don’t think we need to worry about that with you.” Which made her wonder: “Is he telling me I’m never going to get married?”
Quinn applied to graduate school, but she hedged her bets. “There were no women in the faculty at Stanford at that time in the physics department,” she said. “I didn’t see myself there.” She thought she would “apply for Ph.D. programs because good universities don’t offer master’s degrees in physics, but really I’d do a master’s degree and then go take education courses and be a high school teacher.”
Instead, she went on to make seminal contributions to our understanding of basic particle interactions. In the 1970s, she worked with Roberto Peccei on a proposed solution to the strong charge-parity (CP) problem. The puzzle has to do with why a kind of symmetry between matter and antimatter is broken in weak interactions, which drive nuclear decay, but not in strong interactions, which hold matter together. Peccei and Quinn’s solution, known as the Peccei-Quinn mechanism, implies a new kind of symmetry that predicts the existence of an “axion” field, and thus a hypothetical axion particle. Axions have been invoked in theories of supersymmetry and cosmic inflation, and have been proposed as a candidate for dark matter. Physicists are searching high and low for the elusive particle.
Her work on the strong CP problem and other contributions to particle physics have been recognized with prestigious awards including the Dirac Medal, the J.J. Sakurai Prize, the Klein Medal and the Compton Medal. Meanwhile her attention has shifted back to science education. Starting in the late 1980s she led the science education outreach effort at the Stanford Linear Accelerator Center (SLAC), and she later chaired the National Research Council’s Board on Science Education, which developed the framework that led to the Next Generation Science Standards. Quanta Magazine caught up with Quinn at last year’s International Teacher-Scientist Partnership Conference in San Francisco. An edited and condensed version of the conversation follows.
QUANTA MAGAZINE: What was it like entering the field of particle physics in the 1960s?
HELEN QUINN: It was a very exciting time. The thing we now call the Standard Model was just beginning to take shape, and SLAC had just been built at Stanford. In fact, the reason I became a particle physicist is probably because there were so many people around me who were so excited about the science. But I never at any point said, “I’m going to be a physicist. That’s what I want to do.” It just sort of grew on me as I learned more about it.
You did a year of student teaching.
I did my Ph.D. in four years, and it was an interesting piece of work that got noticed. During graduate school, I’d married. My husband was another physicist, and we took postdocs in Germany. Coming back, my husband was offered a faculty position at Tufts, and I said, “Well, if there’s any town in the country where there ought to be another job, it’s Boston because there are seven universities in the Boston area, or probably more.” But I didn’t get a job.
I thought, “OK, I’ll fall back and I’ll be a teacher,” and I took education courses at Tufts and did the student teaching.
Then what happened?
During that semester when I was doing the student teaching, I happened to run into one of my graduate school friends, Joel Primack, who was then a junior fellow at Harvard, and he said, “Why don’t you come talk to us at Harvard sometime?” At that moment, a piece of research came along which was really fundamental to the development of the Standard Model. Gerard ’t Hooft and Martinus Veltman [who shared the 1999 Nobel Prize in Physics] provided a method for calculating the mathematics in gauge theories, which underlie the Standard Model. So I started working with my friend and one other junior faculty member at Harvard, Tom Appelquist, on applying that method to what we call one-loop calculation.
Before the Standard Model, there was a problem with weak interaction theory. You could do the first-order calculation, but the next order (the one-loop calculation) was infinite. So the theory was not well-defined and not stable. We did the first finite one-loop calculation of weak interactions using the new theory. At that point I realized this is drawing me in more than the teaching.
You didn’t like teaching?
I loved the teaching. I hated supervising study hall and the intellectual atmosphere of the high school. So it was not the teaching that put me off as much as it was the intellectual draw of something really exciting going on directly in my field, in my area of interest in physics, that basically was the beginning of the development of the Standard Model. It was an opportunity that I couldn’t turn down.
Later in your career, why did you become involved in trying to fix science education?
After I was elected to the National Academy of Sciences my background in education outreach work meant I was invited to join the Board on Science Education. The opportunity this provided to be involved with science education more broadly was attractive, but more than that it was a chance to learn some interesting things about teaching and learning. As a scientist, if you think you know something without having done any research on it, you probably don’t know. So I said, “Who does understand what’s effective in teaching science?”
There was a study called “Taking Science to School” for which I was part of a committee with people who research learning. I was able to learn how they studied the question: What is most effective in teaching science? That was the beginning of my education about research on learning.
The challenge for me was to understand what the other people in the room were arguing about. At the beginning of that study, I was the physicist, and these were education researchers. And they were having an argument, and I did not know what they were arguing about. I couldn’t discern the differences in their positions because I didn’t know the history.
Later, after the Common Core came along and 47 states adopted common standards in math and language arts, the Carnegie Corporation of New York came to the Board on Science Education and said, “We should be doing this for science, too.” If many states are doing common things in math and language arts, why not think about what they could do in common in science?
You were the chair of the Board on Science Education by that time. What areas of science education did you think needed to improve?
The general conclusion really is embodied in the “Framework for K-12 Science Education” we developed: You have to engage the students in doing things in order for the learning to become meaningful. Just memorizing the knowledge that other people have produced doesn’t really lead to transferable knowledge. The big issue is knowledge that you can apply.
The question is: How do you change learning so that the knowledge becomes much more integrated into the way a person approaches problems outside of school?
What was the biggest challenge for you in developing these standards?
The challenge, but also the fun, of doing it is to try and take a group of people, all of whom have expertise in different areas, and come up with a common view that’s based solidly enough on everyone’s expertise that other people will buy into it and carry it forward. And I think we succeeded with the Framework. Science teachers are generally enthusiastic about the picture we’re putting before them. When I talk to scientists, they’re generally enthusiastic about this way of describing science. So the synthesis works, but achieving it is a group effort. Chairing such a group and bringing it to consensus is a challenging but rewarding process.
And so in some sense, the common view that came out of the Framework became the Next Generation Science Standards.
The standards are based on the Framework, and it helps to read the Framework to understand the intent of them. Standards are, by their very nature, knowledge in pieces. A standard has to be something where you can say: Can the student do that or not?
Essentially, standards are the basis on which you build assessments, and they’re a set of guideposts for teachers and curriculum developers. So standards are in fact not the way to convey the bigger vision. They’re all the little bits and pieces students need to know or be able to do, and in and of themselves, they don’t make sense. Unless they’re built on a bigger vision and unless you have some idea of what that vision is, reading the standards is confusing.
So the Framework is the vision.
The Framework is the vision. The standards are a set of stakes in the ground. If students can do this in third grade, if students can do that in fifth grade, if students can do that in 12th grade, then they have learned sufficient science.
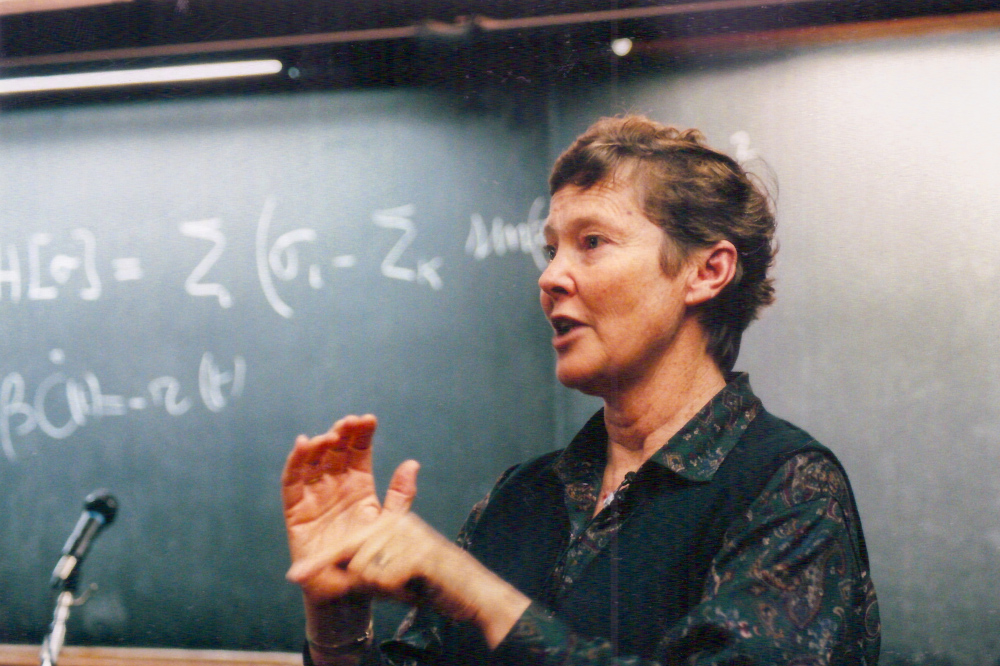
Helen Quinn giving her Dirac Medal lecture in 2000.
Courtesy of Helen Quinn
You describe the Next Generation Science Standards as three-dimensional science learning. What does that mean?
What I mean is that to learn science, you have to learn core ideas from the disciplines of science. [In physical science, these ideas include matter and its interactions, motion and stability, and energy.] But you also have to learn how those ideas were arrived at, what scientists do, the practices of science, and the practices of engineering, both in order to understand the nature of science and in order to engage in those practices to make the learning your own. That is a second dimension to science learning. And finally, the third dimension is that there are some big concepts which you need in order to know where you’re going and to know which kinds of questions to ask when you’re looking at a new problem. These are concepts such as the fact that explanations in science are about cause and effect mechanisms, or that, in order to decipher these mechanisms, it is useful to define and make a model for the system in which a phenomenon occurs. And those big concepts are very often not taught. Students are sort of expected to get them as a side effect of doing things over and over again.
And you call that third dimension “crosscutting.” Is that meant to imply that you’re cutting across different disciplines?
Right. These are the concepts which apply whether you’re doing physics, chemistry, biology, earth science or any other area of science. These will be useful lenses to look at a problem with.
Isn’t it harder to assess whether students have learned crosscutting concepts and the process of science?
Queensland and other states in Australia in fact do this. Some part of the state assessment is an external exam, but part of it is performance assessments in the classroom that are graded by the teachers. First of all, this approach trusts teachers as professionals, but secondly, it has a cross-check system. If there’s an imbalance between the external testing part and the teacher’s grading of the in-class part, then inspectors come and they watch. So there’s a whole structure developed around having the teachers be part of a professional system and monitoring that system.
In the U.S., we have adopted a system of drop-in-from-the-sky external testing where the teachers play no role in it. That’s actually a very inefficient model because the teachers know a lot more about the students than any drop-in test can discover. Assessments that drop in from the sky are designed to be cheap and to be scored by machine; it’s very limited. Mostly it just tests what has been memorized. And having our entire education system designed to have students be able to get high scores on those tests is counterproductive. It drives all the wrong behaviors into the classroom. So we need new types of testing tasks to test whether students have achieved these new three-dimensional standards and to drive the teaching and learning behaviors that we know are more productive.
Now that the standards are out there, what are you focusing on?
My term on the Board on Science Education is up, so I no longer have that particular platform to work from. I go where I’m invited to give talks, to sort of wave the flag and talk at the county level or the state level about what the standards are and why they were developed, and to help people understand how to implement them.
When you talk to teachers, what advice do you give them about making science more interesting for students?
There are two things: first, building learning around observable events or phenomena. And, second, getting students engaged with a question before you give them the answer. We all get much more interested in things if we have a question about it than if somebody is telling us something that we haven’t any reason to know we want to know.
What’s the endgame?
I want educated people. I want citizens who can look at a problem in their community and think like a scientist about the part of the problem that is science. I want high school and college graduates with capabilities that employers want, whether they come from well-educated families or not. I want them to be able to take on a problem and solve it because that’s what employers are looking for. They want you to be able to work on a team; to be given some information, interpret it and use it; to not have to be told: “This is what you do tomorrow.” And all of those things require something more than just being able to repeat back what you were told. So that’s where I’m going. I think it’s a huge equity issue.
This article was reprinted on Wired.com.